I am sure that, at least once, every single one of you has thought about how the bird is able to survive at such high heights for days on end while undertaking such strenuous exercise! Naturally one turns to the respiratory system, and in this blog post, I will offer some reasons as to how our winged friends are able to achieve such feats. I hope you enjoy!
360 million years ago, organisms moved from the ocean to shallower waters, eventually reaching land. The last known common ancestor between the bird and the mammal, the amniote, dates back to 320 million years ago. Since this vital period in the development of life on earth, birds and mammals have evolved in their own fashion, through different genetic mutations and selection pressures, to become different classes. Although their organ systems are similar in many ways, the stark difference between the two is the way in which their lungs are physiologically different, where gas exchange is noticeably more efficient in the bird lung. This allows birds to fly up to 37,000 feet above sea level (TMW, n.d.) in the case of certain species, whereas the average human passes out around 15,000 feet above sea level due to a lack of oxygen. This blog will explore the human and bird lungs, explaining why birds have the capability to survive at such high altitudes.
In order for the later sections of this article to be understood, one must first understand the similarities between the human lung and the bird lung. Firstly, both humans and birds have similar breathing organs, where they both have nostrils, a nasal cavity, a larynx, a trachea, a bronchus and a sternum (Answers 2012). Furthermore, they both have two lungs that are connected to a trachea. This shows that the surrounding organs remain the same, suggesting that it must be the physiological aspects of the lungs of the two animals that prove to be the difference between oxygen level consumption. This shows the vast difference between human and bird lungs.
The physiology of the bird lung is wondrous, allowing it to fly at such high elevations, which leaves scientists pondering as to where the evolution of the human lung went 'astray'. One of the key factors for efficient oxygen consumption of the bird lung is the mechanism of lung ventilation. In comparison to the human lung, the bird lung does not inflate or deflate but rather stays at a constant volume. This means that the air sacs which surround the lungs, which are inflated and deflated in a complex sequence, cause the air to move in and out of the lungs during inspiration and expiration of the nostrils. This is in comparison to the mechanism of human lungs, which change volume themselves due to decreased or increased air pressure - a much more inefficient method of gas exchange. Furthermore, the alveoli in human lungs are replaced by parabronchi: thin-walled, tubular structures, which are covered by a rich supply of capillaries, and carry air from rear-end sacs to front-end sacs between secondary bronchi (Greij 2014).
In addition, the volume of the respiratory system of the bird is up to double that of mammals of similar size (Britannica, n.d.). This is due to the large surface area of the numerous air sacs that surround the relatively small lung, where each bird lung passes on to paired abdominal air sacs, which also give rise to secondary bronchi supplying other air sacs. Tertiary bronchi penetrate the lung mass and lead to fine air capillaries, which are analogous to alveoli, where their walls contain blood capillaries connected with the heart. Although not directly involved in gas exchange, the thin-walled air sacs direct airflow in one direction. This leads to the next major difference between bird and human lungs.
The speciality of the air sacs that make up the majority of the respiring system of the bird is that they accommodate a continuous one-way flow of air through the lungs, both during inspiration and exhalation. Air sacs expand during inhalation and compress during exhalation, leading to two breaths needed for air to fully pass through a bird’s respiratory system. During inspiration, air is pulled into the posterior air sacs (coming off the back of the lungs as shown in Figure 1), which is then pushed into the lungs as air sacs compress during exhalation. During the second breath, air is drawn into the anterior sacs (coming off of the front of the lungs as shown in Figure 1), which is then exhaled as the air sacs compress (Eastern Kentucky University, n.d.). This creates the one-way back-to-front flow through the lungs and allows birds to have a constant stream of oxygen to fulfil the metabolic needs of flight.
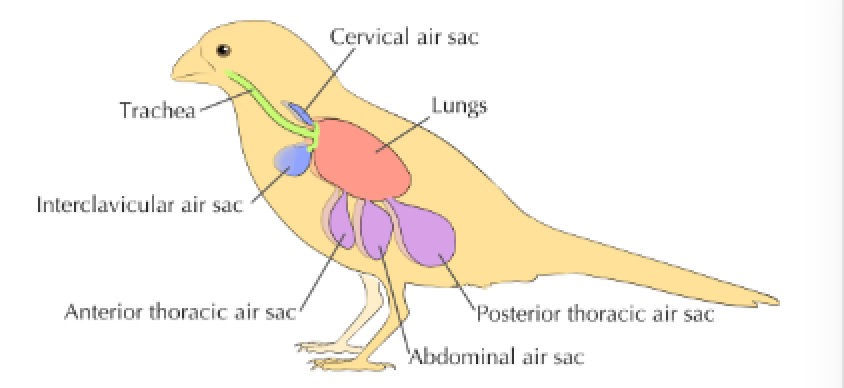
Figure 1 - The Avian Respiratory System
Furthermore, ventilation of birds increases greatly during flight, due to the precise synchrony between breathing and wing motion, where the peak of expiration occurs at the downstroke of the wingbeat, as well as an increased number of breaths per minute. This leads to the ventilation of certain birds, such as pigeons, increasing by 20-fold during flight.
In comparison to the highly effective unidirectional system of gas exchange in the bird lung, the bi-directional pattern of airflow in mammals causes further problems - due to the fact that the airflow is not in one direction, the lungs can never fully empty with each expiration. In contrast, approximately 3 litres of air remain in the lung as a ‘resting gas volume’, while each inhalation only takes in approximately 0.5 litres of air. This means that convection, fluid flow, cannot push the inhaled air to the outer limits of the lung where some alveoli are located, as shown in Figure 2. Although the air can enter the lungs through inhalation, a combination of both convection and diffusion is required for the oxygen-rich inhaled air to reach the peripheral areas of the lung. This requires large terminal air spaces to allow the mixing of newly inspired air with air already in the lung. This shows the inefficiency of bidirectional flow of air, and how with unidirectional flow, higher rates of gas exchange can occur while taking up less volume of the body.
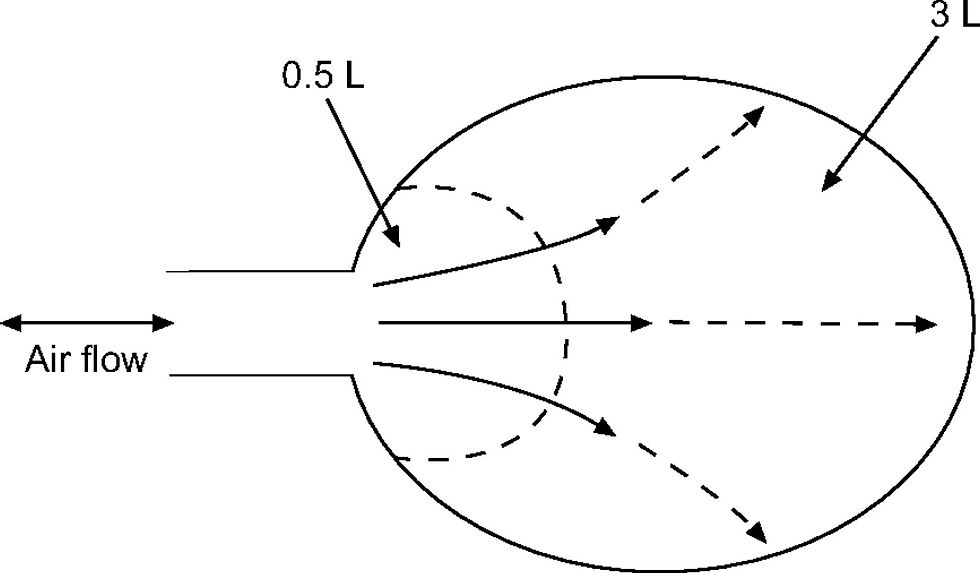
Figure 2 - Pattern of Ventilation in a Mammalian Lung
Furthermore, the result of a low volume of gas being inspired into an already large pool of gas leads to low alveolar, and therefore arterial oxygen pressure in comparison to the pressure of the inspired gas. In the human respiratory system, the alveolar oxygen pressure is approximately 13.3 kPa, in contrast to the inspired gas oxygen pressure of 20 kPa (West, Watson, and Fu 2007). This decreases the efficiency of oxygen intake in the human lung, in comparison to the unidirectional flow of the bird lung, which greatly reduces this problem.
It has also been proven that the bird lung is efficient partly due to its extremely thin blood-gas barrier, so much that some birds have thinner barriers than any other mammals. The mean thickness of some birds’ barriers can reach <0.1 micrometres, compared to the mammal with the thinnest barrier, the Etruscan Shrew, sitting at 0.33 micrometres. In addition to this, papers have shown that in addition to the total barrier of the bird being very thin, the interstitium, which is responsible for the barrier's strength, is also extraordinarily thin (Fu 2006). Although this is highly paradoxical, as being capable of extreme physical activity while having a very thin ECM, which is exposed to large mechanical stresses during flight, is highly peculiar, it is currently thought that epithelial struts help maintain the integrity of the capillary walls. The capillary wall on one side of the human alveolus is thin, while the other is thicker due to type 1 collagen cable attached, as shown in Figure 3b, to help maintain the integrity of one long alveolar wall, which is generally poorly supported. In contrast, the bird blood-gas barriers are very thin and uniform, as shown in Figure 3a, as pulmonary capillaries in the bird lung are embedded in a honeycomb-like structure of air capillaries, and there is no long alveolar wall that requires a type 1 collagen cable for support. In fact, the blood-gas barrier in the bird lung is thinner than the thin side of the human capillary wall. A shorter diffusion distance in the bird lung leads to quicker diffusion of oxygen, therefore increasing the efficiency of gas exchange once again.
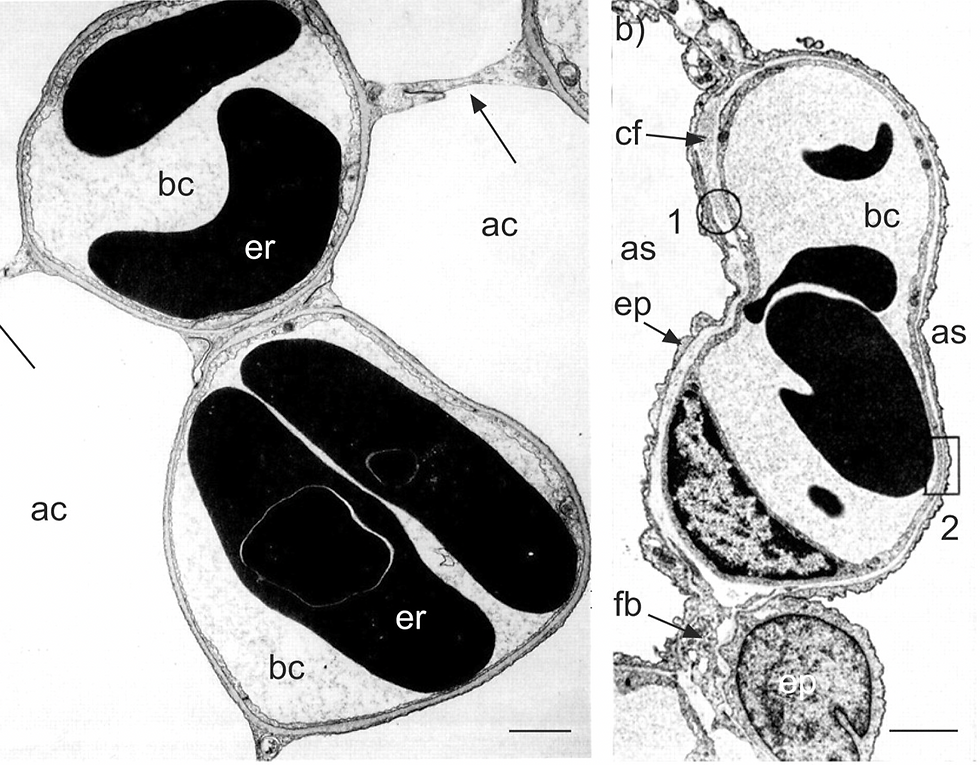
Figure 3 (left) - Electron Micrograph from Blood Capillaries in a Bird Lung
(right) - Electron Micrograph from Blood Capillaries in a Mammalian Lung
In conclusion, there are many different ways in which the physiology of the bird lung increases the efficiency of oxygen intake including the higher surface area of voluminous air sacs; unidirectional airflow in the lungs; and thin blood-gas barriers. This allows the bird to fulfil its metabolic needs during flight at such high heights, where oxygen partial pressure is very low and explains why birds are able to survive at such high altitudes. This blog has proved the efficiency of the avian lung while highlighting the inefficiency of the human lung and provides the question as to where the evolution of the human lung went astray. This however can be extended fully in another blog - leave a comment if you would like to see something on this!
Below is a list of the articles that I used in my research for this blog, if you want to research this topic more in depth! Thank you for reading and I hope you enjoyed!
Britannica. n.d. “Respiratory system - Birds | Britannica.” Encyclopedia Britannica. https://www.britannica.com/science/respiratory-system/Birds.
Eastern Kentucky University. n.d. “Avian Respiration.” Bird Respiratory System. http://people.eku.edu/ritchisong/birdrespiration.html.
Fu, Zhenxing. 2006. “The honeycomb-like structure of the bird lung allows a uniquely thin blood-gas barrier.” PubMed. https://pubmed.ncbi.nlm.nih.gov/16431166/.
Greij, Eldon. 2014. “How birds breathe.” BirdWatching Magazine. https://www.birdwatchingdaily.com/news/science/eldon-greij-describes-amazing-way-birds-breathe/.
TMW. n.d. “Top 10 Highest Flying Birds In The World.” The Mysterious World. https://themysteriousworld.com/10-highest-flying-birds-in-the-world/.
West, J. B., R. R. Watson, and Z. Fu. 2007. “The human lung: did evolution get it wrong?” ERS publications. https://erj.ersjournals.com/content/29/1/11#F4.
Comments